The quest for commercial-performance electronics in space – Military and Aerospace Electronics
By John Keller – June 21, 2023 – Military and Aerospace Electronics
The sharply growing number of space applications today is driving innovation in radiation-tolerant and radiation-hardened electronics for tasks ranging from signal processing, digital conversion, power management and control, and solid-state data storage.
The primary trend is to design rad-hard and rad-tolerant electronics affordably enough and with sufficient performance to accommodate the widest possible range of space missions, from short-duration low-Earth-orbit commercial small satellites to deep-space missions such as exploration of Jupiter and its moons.
That’s not an easy task. Rad-hard electronics parts historically have been expensive and lag in performance behind their commercial counterparts. For today’s booming space industry, that’s no longer acceptable.
Today’s rad-tolerant and rad-hard electronics parts must offer performance comparable to state-of-the-art commercial components, must offer at least some level of resistance to space radiation, and offer unprecedented levels in small size, weight, power consumption, and cost (SWaP-C). Achieving these goals is among the biggest challenges in the space industry today.
Rad-hard design
On the high and somewhat-expensive end, some rad-hard suppliers are designing parts specifically to operate reliably long term in radiation environments like space, high-altitude aviation, and the nuclear power industry. “Our enabler is the radiation-hardened by design methodology,” explains Jens Verbeeck, CEO of rad-hard parts supplier Magics Technologies nv in Geel, Belgium.
“We use multiple technology nodes from commercial foundries where they also build state-of-the-art components,” Verbeeck says. “We have developed libraries
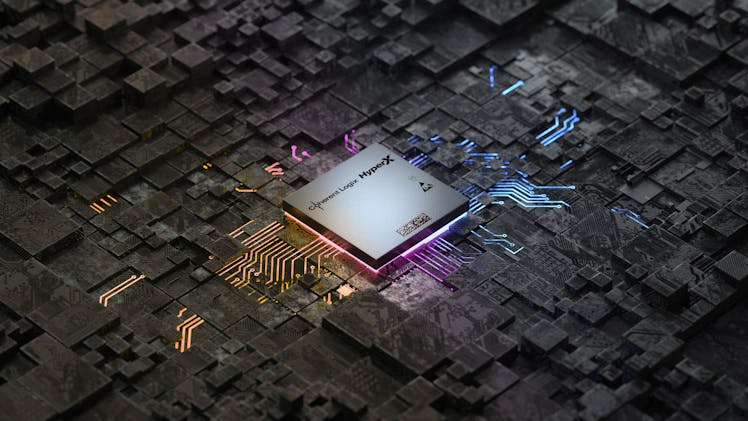
and simulations with a design library, and we can make state-of-the-art components that have unmatched performance. We are working on AI [artificial intelligence] accelerator cores for next-generation optical payloads to be used in space, which are used for mapping everything that happens on Earth.”
In addition to starting with commercial designs, Magics also can provide custom designs for radiation-hardened electronics. “We can make the chip really bottom-up,” he says. “By knowing the specifications of our customer, we make the schematics and lay out the chip, which then goes to a commercial foundry. We can use any foundry; that’s the neat thing about it.”
Magics’ Verbeeck points out that his company’s design approach “is not based on upscreening of COTS [commercial off-the-shelf] parts. It is based on state-of-the-art components, and we also can bring that semiconductor evolution to the space domain.”
VPT Inc. in Blacksburg, Va., takes a different approach, and relies on testing commercial parts to determine which ones could meet the needs of many different space missions, depending on the radiation environment.
VPT specializes in radiation-hardened power control and conditioning parts for space applications in a wide variety of radiation environments. “There are lots of ways to deal with radiation. Shielding is one, but you can’t completely get rid of the problem with shielding,” says Leonard Leslie, vice president of engineering at VPT.
“The solution involves parts selection, and determining which parts can withstand the radiation with parts testing. we have several lines of our power converters that are designed for the space environment.
Using commercial fabs
Apogee Semiconductor in Plano, Texas, also relies on using commercial semiconductor technology as a baseline for their space components designs. “We have a rad-hard process to fabricate in commercial foundries to develop lower-cost components,” says Anton Quiroz, CEO and founder of Apogee Semiconductor.
“Our process works with commercial foundries to take their standard circuits and improve their radiation performance by an order of magnitude,” Quiroz explains. “We leverage equipment the fab already has to develop a transistor with the performance of a standard transistor. We proved our process. We then used the process and IP blocks to develop a pulse width modulation [PWM] controller for power management for different voltage domains. It is an hybrid analog and a digital controller.”
Apogee also can radiation-harden plastic encapsulated semiconductors for space applications to provide high-performance parts at relatively ow costs. “Our main release is in plastic packages,” Quiroz says. It offers better performance and is less expensive. These parts tend to be smaller and weigh less.”
The company also relies on extensive parts testing and upscreening based on statistical process control monitoring. “This helps us take some tests out of our screening flow and really reduce the cost of the components,” he says. “We look at statistics of our products from lot to lot, and identify outliers.”
Offering one of the widest selections of radiation-hardened and radiation-tolerant parts is Microchip Technology Inc. in Chandler, Ariz. “we have rad-tolerant enhancements not designed originally for space,” says Nicolas Ganry, senior product manager for aerospace and defense at Microchip. “On top of that, we have rad-hard devices especially designed for aerospace and defense and space market.”
Ganry explains that Microchip experts seek to avoid expensive complete device redesigns to qualify them for space in some, if not all, radiation environments. “If we can introduce some radiation hardened designs without redesigning everything it is better for performance and time to market.” He says a large scope of radiation-tolerant devices for aviation and space can start from parts designed originally for commercial or automotive uses.
So called “new space,” which involves the emergence of private space industry companies involved in applications such as private launch endeavors, small satellite constellations, or sub-orbital tourism, is driving innovation in radiation-tolerant and radiation-hardened electronics.
“Players in new space are starting to think in a different way,” Ganry explains. “They are using commercial devices as much as they can, challenging the level of the component according to the missions they have to manage. Radiation impact is linked to duration. This involves not only technological expertise, but also new levels of risk management. If you have a large satellite constellation, you can take the risk of losing one, but with one large satellite in geosynchronous orbit, you can’t take risks with the components.”
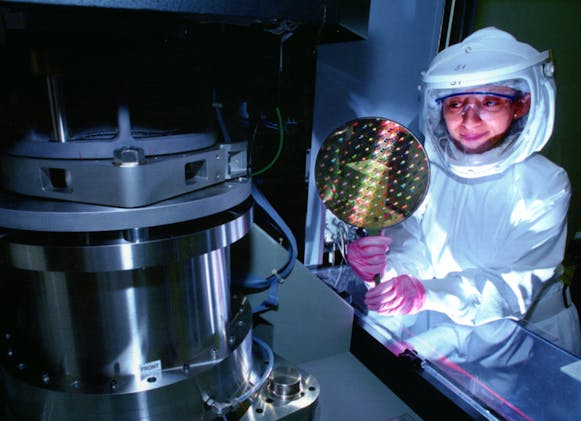
Chip fabs still are manufacturing processors and other electronic components specifically for radiation environments in space, but commercial satellite constellations are encouraging chip makers to upscreen commercial chips for specific environments.
Just how well electronic parts resist the effects of space radiation is up to the customer, Ganry explains, “according to the profile of the mission, they can leverage the hardness of the device. We don’t drive the choice of the customer; we offer what we have, and then it is up to each customer to select the right device for the application.”
Microchip often can select or tailor rad-hard and rad-tolerant parts to specific target environments “We can take a commercial part, raise it to radiation-tolerant level, and put it in a ceramic package, and qualify it for space,” he says. “For some we can keep the plastic package and add some additional qualification beyond automotive-grade where we improve some life tests, impose some qualification reports that we propose around our rad-plastic flow for our customers. It is an additional solution. On top of traditional ceramic solution.”
Rad-hard solutions
Coherent Logix Inc. in Austin, Texas, offers the HyperX family of radiation-hardened software-defined microprocessors. One of the family’s early models, the hx2100, has seen design-ins in a variety of space projects, says Michael Doerr, Co-CEO and CTO at Coherent Logix.
“Satellite payloads, communications, or critical control systems in a spacecraft historically have been built stove-piped with one function and one use case,” Doerr explains. “That’s not the case anymore. Satellites are multi mission capable, and evolve their missions over time, and need reliability. “Today you Need a fully programmable solution up there. Traditional solution is FPGAs, but are high power, and are not friendly to reprogram from a mission standpoint. To build an ASIC would be very expensive. Our solution provides the performance of an ASIC or FPGA, but has the power consumption of an ASIC. Now getting the best of both worlds. Used to be a pure hardware solution and now is a software solution.
The Coherent Logix HyperX family of programmable space processors offer “a fully software-defined platform for communications, video imaging, AI, and adaptive processing on the satellite, with software-defined networking, and active cyber security. Now you have the ability to do that.”
The HyperX family offers the ability to do on-orbit processing at data plane rates,” Doerr says. “It’s an absolute game-changer with what we can do with cyber security and software-defined networking. The HyperX family, he says is a different type of fabric. “Some call it polymorphic, others call it multiprocessor fabric. It’s a blend of all of those. Its heritage is looking at biologically inspired systems of how our brain works. It is digital neuromorphic processing — a unique architecture that is now being accepted into the marketplace.”
HyperX can be designed for low-, medium-, geosynchronous orbits — for short- and long-duration missions, Doerr says. The company’s latest offering is the radiation-hardened HyperX: Midnight system on chip (SoC) processor for commercial space applications like communications satellites. HyperX: Midnight offers as much as four times the computing throughput, half the power consumption, and a 40 percent lower price compared to leading radiation-hardened field-programmable gate arrays (FPGAs), company officials say.
Software-defined space processors
This enables greater capabilities to be packaged in smaller satellite bus sizes to help lower launch costs and accelerating industry growth. HyperX: Midnight also brings software-defined operations, C-programmability, and simplified software debugging to commercial space. Combined with Coherent Logix’s HyperX Studio design suite, these features offer full on-orbit re-programmability, so improvements can continue long after launch. HyperX SoCs support market solutions across several sectors, including consumer electronics, 5G mobile infrastructure, video/broadcast infrastructure, machine vision, sensor processing, and artificial intelligence.
Renesas Electronics America in Palm Bay, Fla., offers radiation-hardened power conditioning and control components for space applications. Driving power innovation for space are increasingly power-hungry components for space systems such as the Versal field-programmable gate array (FPGA) from Xilinx Inc. in San Jose, Calif. “The Xilinx Versal FPGA is getting a lot of traction, yet with its feature set it requires so much more power, so you need to design new solutions around it,” says Josh Broline, senior director of product marketing applications at Renesas.
“We have a full solution set for Versal and every other FPGA out there today,” Broline says, explaining that satellite electronics designers are minimizing the amount of data uplinks and downlinks to Earth today, and increasingly use on-board processing that the Versal offers.
“They have to do real-time acquisition of fast-moving projectiles, and make decisions in real time. That’s the real deal when it comes to applications,” Broline says. We also are pushing the envelope in precision A-D converters to optimize the resolution and what you can get off of sensors aboard a spacecraft or rover.”
Demands for on-satellite data processing are expected to grow into the foreseeable future. “At the systems level, what happens in the optical communications area for satellite-to-satellite communications, and satellite to ground? “We need to plan for multi-orbit strategies and capabilities, and to move and adapt to the customer’s needs.”
The future will bring new generations of reconfigurable and flexible satellites, software-defined communications and functionality, and similar on-orbit capabilities. “I expect innovation to come out of system level, and that will pull the component suppliers to meet customer needs.
Influential space programs
Microchip is involved in the High-Performance Spaceflight Computing (HPSC) processor project of the U.S. National Aeronautics and Space Administration (NASA) Jet Propulsion Laboratory in La Cañada Flintridge, Calif. Microchip is developing a space processor that will provide at least 100 times the computational capacity of current spaceflight computers.
Microchip will build the HPSC processor over three years, with the goal of employing the processor on future lunar and planetary exploration missions. Microchip’s processor architecture will improve the overall computing efficiency for these missions by enabling computing power to be scalable, based on mission needs. The work is under a $50 million contract, with Microchip contributing significant research and development costs to complete the project.
“We are making a joint investment with NASA on a new trusted and transformative compute platform that will deliver comprehensive Ethernet networking, advanced artificial intelligence and machine learning processing, and connectivity support while offering unprecedented performance gain, fault-tolerance, and security architecture at low power consumption,” says Babak Samimi, corporate vice president for Microchip’s Communications business unit.
“We will foster an industrywide ecosystem of single-board computer partners anchored on the HPSC processor and Microchip’s complementary space-qualified total system solutions to benefit a new generation of mission-critical edge compute designs optimized for size, weight, and power,” he says.
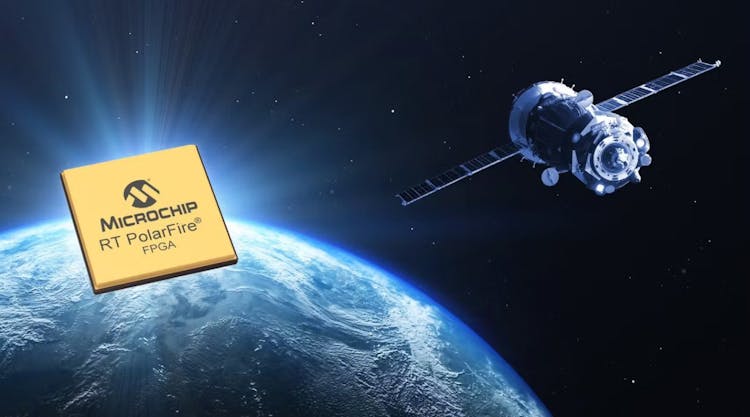
Current space-qualified computing technology is designed to address the most computationally intensive part of a mission, which leads to overdesigning and inefficient use of computing power. Microchip’s new processor will enable the device’s processing power to ebb and flow depending on current operational requirements. Certain processing functions can also be turned off when not in use to reduce power consumption.
“Our current spaceflight computers were developed almost 30 years ago,” says Wesley Powell, NASA’s principal technologist for advanced avionics. “While they have served past missions well, future NASA missions demand significantly increased onboard computing capabilities and reliability. The new computing processor will provide the advances required in performance, fault tolerance, and flexibility to meet these future mission needs.”
The U.S. Space Force has kicked-off a program to design next-generation radiation-hardened non-volatile memory chips for future military applications in space in the Advanced Next Generation Strategic Radiation hardened Memory (ANGSTRM) project. The U.S. Air Force Research Laboratory’s Space Vehicles Directorate at Kirtland Air Force Base, N.M., issued an ANGSTRM solicitation on behalf of the Space Force last January to develop a strategic rad-hard non-volatile memory device with near-commercial state-of-the-art performance by using advanced packaging and radiation-hardening techniques with state-of-the-art commercial technology.
Advanced rad-hard memory
Advancing strategic rad-hard non-volatile memory technologies is critical to support strategic missiles, missile defense, and military space systems, researchers say. Non-volatile memory devices retain their data even when they lose power. Ideally, the DoD would have access to non-volatile memories with the performance and density of commercial state-of-the-art devices; unfortunately today’s commercial technologies are not able to withstand the radiation and thermal environments where the military deploys systems. Many military systems, moreover, must use trusted on-shore electronics manufacturing.
Space Force researchers are interested in combining radiation hardening to state-of-the-art CMOS and memory technologies to scale density beyond the levels of a single chip, and create qualified strategic rad-hard non-volatile memory for use across military space and strategic systems.
Researchers want to industry to develop rad-hard memories with monolithic memory densities of 4 to 16 gigabits, and with multichip module densities of 32 to 128 gigabits that will last without refresh for 10 to 15 years. Memory devices should operate with no more than 10 milliwatts of power, with 1 milliwatt performance preferred. Total standby power requirements are no more than 10 milliwatts, and as little as 10 milliwatts. These devices should operate in temperatures from -40 to 125 degrees Celsius, and eventually down to temperatures of -55 C. Companies interested were asked to submit white papers by February.
Last October the U.S. Defense Advanced Research Projects Agency (DARPA) in Arlington, Va., issued a broad agency announcement for the Space Power Conversion Electronics (SPCE) project to develop radiation-tolerant high-voltage transistors with high performance and small size to enable compact, high-conversion-ratio point-of-load power converters for space applications.
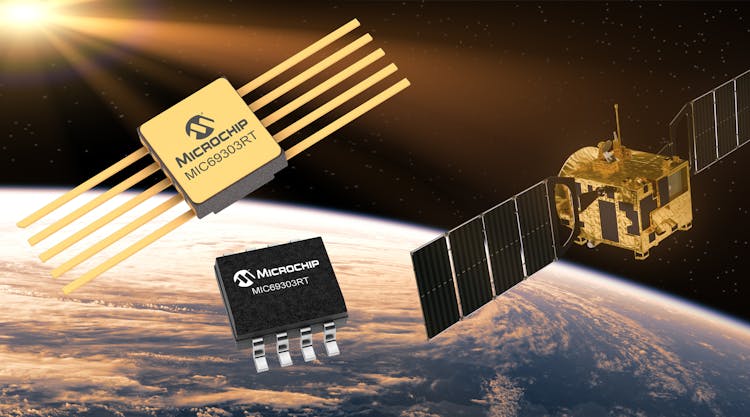
SPCE seeks to develop high-performance integrated radiation-tolerant high-voltage transistors that perform better than today’s advanced wide bandgap semiconductor (WBGS) high-voltage devices for non-radiation environments. Researchers seek to demonstrate a 48 V-to-1 V radiation-tolerant point-of-load converter with better than 85 percent power at 50 amps of output current, with power density greater than 500 Watts per cubic inch.
To do this, researchers have laid out two key technical challenges: achieving a high-performance high-voltage transistor that is radiation-tolerant; and achieving a low-loss, high-voltage integrated circuit that is radiation tolerant. Today’s most advanced space point-of-load systems use discrete radiation-hardened silicon lightly doped-drain MOSFET (LDMOS) transistors, which limit the overall point-of-load efficiency to less than 60 percent. To avoid single-event burnout, these LDMOS transistors operate at a maximum voltage substantially lower than the device breakdown voltage, which leads to degradation in performance. The SPCE project focuses on two technical areas: radiation-tolerant high-performance high-voltage transistor and high-efficiency point-of-load DC-DC converter; and fundamental device exploration. Companies interested were asked to submit proposals by last December.
https://www.militaryaerospace.com/computers/article/14293820/space-electronics-radiation-hardened